TECHNICAL REPORT
Grantee |
RMIT University
12.08.12, School of Engineering RMIT University Melbourne, VIC 3000 Australia
|
Project Title | Network Remote Powering through Quasi-Passive Reconfigurable Nodes |
Amount Awarded | USD 20,000 |
Dates covered by this report: | 2019-12-09 to 2021-12-20 |
Report submission date | 2022-05-09 |
Economies where project was implemented | Australia |
Project leader name |
Ke Wang
|
Project Team |
Kandeepan Sithamparanathan
Yitong Wang
|
Project Summary
This project investigated the remote powering of Internet using the quasi-passive network nodes, where the power required for the providing Internet in remote network nodes without local power supply is remotely provided optically. The pump light for remote powering of network nodes is transmitted using the same data transmission fibre to reuse the existing network infrastructure. The major objectives of the project are to investigate, develop and optimize the network node and the remote power scheme, to increase the scalability, traffic handling capability and efficiency, via both theoretical study and numerical simulations. The project was originally planned to be completed in 12 months. However, due to the interruption of COVID-19, the university campus was locked down for a prolonged period and no access to the university was allowed, This interruption resulted in delays in the project, and the project was completed at the end of May, 2021 (the research paper was published in mid December, 2021). Although the project has been significantly affected by the COVID-19 situation, the project team managed to adjust the plan slightly to focus more on the theoretical study, modelling and analysis during this period. The main activities conducted in this project include: The model of individual components in the node has been completed; The signal propagation model in the network node has been built; The theoretical model of the remote powering scheme has been established; The remote powering scheme based network node has been analyzed theoretically and through comprehensive numerical simulations; The complexity of the remote powering scheme based network node has been analyzed; The impact of the opticam pump on the signal transmission in fibre has been studied; The impact of different data modulation formats in remotely powered network node has been investigated; The multiple optical pumps scheme has been proposed and studied to further increase the availability of power to nodes without local power supply, and two different configurations of the multiple optical pumps scheme have been analyzed and compared; Large-scale optical networks with the proposed quasi-passive network nodes and remote powering technique have been simulated, and the statistical performance has been investigated.
Table of contents
- Project factsheet information
- Background and justification
- Project Implementation
- Project Evaluation
- Gender Equality and Inclusion
- Project Communication Strategy
- Recommendations and Use of Findings
- Bibliography
Background and Justification
Broadband networks have been widely deployed around the world, and optical technology is the main stream to provide data connections, mainly due to the large bandwidth and low loss advantages of optical fibres. Compared with the core network, the access network is more challenging due to the large number of users and the wide span of geographical areas that need to be connected. Providing broadband Internet connections in remote areas (i.e., rural areas) can be more difficult than that in the urban areas, and one key reason is possible lack of local power supply for critical network nodes (i.e., off-grid areas), which provide the data switching, network reconfiguration, and traffic routing functions. Because of this limit, from the user and community point of view, Internet access, especially high-speed access, in a large number of remote areas is largely unsatisfying. This limit also makes the network design more challenging and costly, from the operator perspective.
The limited access to high-speed Internet in remote areas have significantly impaired the living standards of disadvantaged people in remote communities. One particular example is the remote education need imposed by the recent widespread lockdowns worldwide (including Australia) due to the COVID-19 pandemic. For high quality remote online education, high-speed and reliable Internet is a necessity. Whilst such access is mostly available in urban areas, students in rural areas are more severely affected and disadvantaged. There are many more similar examples, such as remote health care and daily entertainment (e.g., high-definition video on demand).
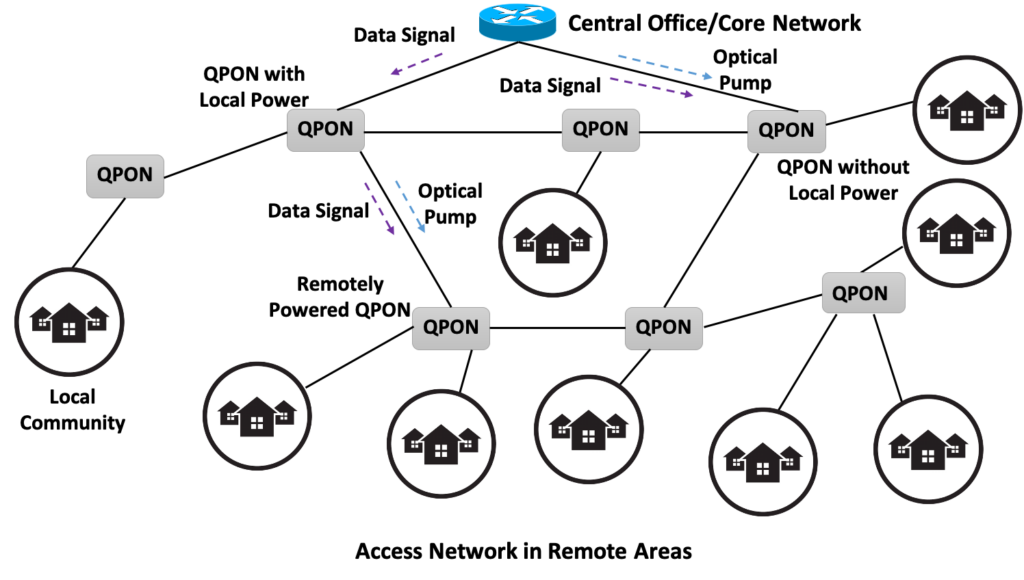
To solve this problem, we study and use innovative quasi-passive network nodes in this project. Such nodes have low power consumption – actually no power consumption during static network operations. Since the network is mostly operated under relatively static conditions, the power need of remote network nodes for data switching and routing or link reconfiguration can be significantly reduced. More importantly, these remote nodes can be remotely powered, where the power required to operate the remote network node can be transmitted in the same data transmission fibre as an optical pump, as shown in Figure 1 above. The remote powering will be realized using optical pump signals, and they can be provided directly from the central office or from the neighbouring nodes with local power supplies. Such new network powering scheme can eliminate the local power supply requirement in the remote nodes without access to local power supplies, and hence, the network infrastructure and access in remote areas can be improved significantly.
In this project, we conduct detailed and in-depth study of the quasi-passive network nodes and the remote powering technique. In particular, we focus on both the positive and the negative aspects of this approach to provide a complete technical analysis. From the positive side, power can be delivered to remote network nodes without local power supply, and hence, the network availability in remote areas can be improved substantially. However, there is also negative side associated with this technique, which is mainly caused by the adverse effects when the strong optical pump and the data signal are transmitted together in the optical fibre. Such negative impact can significantly degrade the performance of the data signal and eventually leads to the failure of data transmission. This project looks at both sides to find a balanced and optimized technical option, which can enable the remote powering of network nodes and at the same time, minimize the impact on the data transmission. Through this way, this project provides a promising technical option to satisfy the network access requirements in remote areas.
Project Implementation
This project aims to use technical innovation to enable better Internet connections and access in remote areas. As described in the Background section, we study the quasi passive optical node (QPON) with remote powering capability to eliminate the requirement of local power supply in network nodes. The project is divided into 3 stages. The target of stage 1 is building the theoretical framework of QPON and the remote powering scheme to understand the fundamental capabilities and limitations. Stage 2 aims to investigate the remote powering schemes in detail through theoretical studies and comprehensive numerical simulations. Although the project is affected by the unpredictable COVID-19 pandemic, we have completed stages 1 and 2 successfully. The detailed project progress is as follows:
Stage 1
1. Framework of QPON
According to the availability of local power supply, two types of QPON can be used to provide high-speed and reliable Internet access to remote areas: (1) type 1 – QPON with local power supply; and (2) type 2- QPON without local power supply.
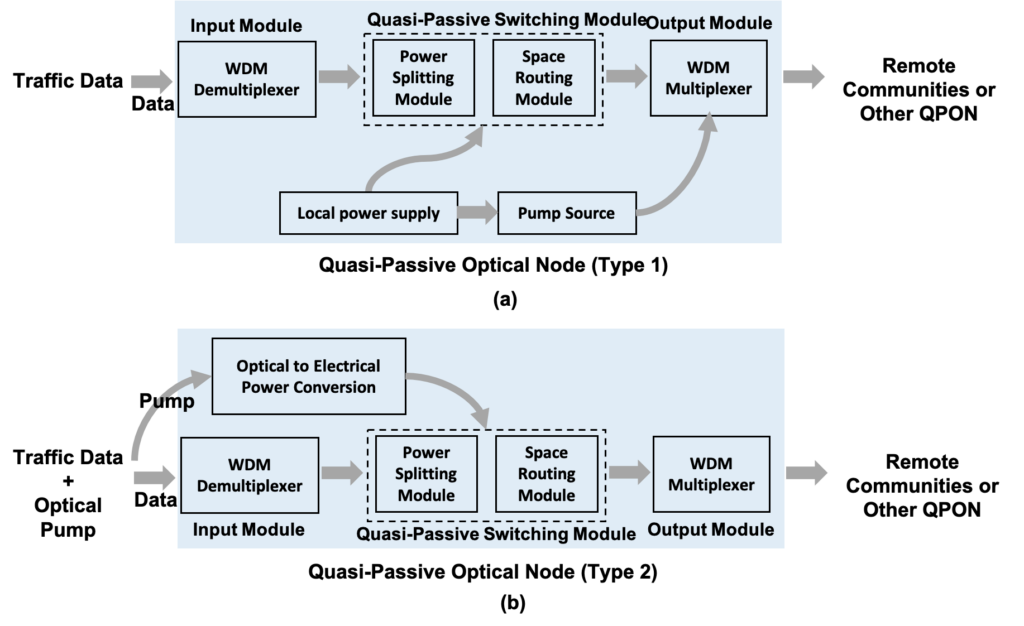
In the type 1, which is shown in Figure 1 (a), since local power supply is available, no remote powering is needed and only the traffic data is transmitted from the previous network node or the central office. In addition, the type 1 QPON will also be equipped with pump light sources to provide remote powering to neighbouring nodes which do not have access to local power supply. On the other hand, in the type 2 QPON, remote powering is required due to the lack of local power supply. The general structure of type 2 QPON is shown in Figure 1(b). Both traffic data and optical pump are transmitted from the previous network node or the central office. The optical pump is separately from the traffic data upon entering into the QPON. The separated optical pump is then converted to electrical power to provide the energy needed to operate the network node.
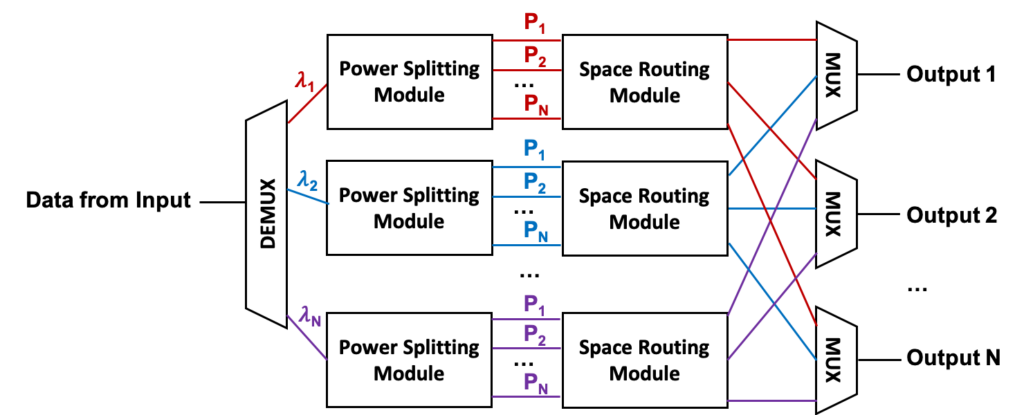
For both types of QPON, the function for data traffic is the same: routing the data traffic to the required output, which can be connected to the next network node or the final destination (i.e., end users). Here we have included the wavelength multiplexer (MUX) and demultiplexer (DEMUX) in the data traffic path to enable the compatibility with the access network using the wavelength division multiplexing (WDM) technology, which has been widely accepted as the future solution to further increase the network speed and capacity. To route the data traffic to the required output, we use the quasi-passive manner to reduce the power consumption, which means no power consumption if the connection from the input to the output remains unchanged. The general principle of such quasi-passive data traffic routing is shown in Figure 2, which consists of the power splitting and the space routing parts. The power splitting part divides the signal to multiple parts if the signal needs to be passed to multiple outputs, and the space routing part guides the divided signals to the required destination outputs. Through this way, flexible and reconfigurable connections of data traffic can be realised. The power splitting part of the QPON is achived by using optical power splitters and the space routing part is reallised using the optical latching switches (OLSs).
Based on the key components inside the two types of QPON, we have established the theoretical framework for analyzing the capabilities and limitations. Two types of major impairments exist inside the QPON to the data traffic. The first one is the loss induced internally, and in the framework we model each key component using the corresponding transmission efficiency. The second major impairment is the crosstalk, which is caused by the imperfect filtering of data signals with different wavelengths and/or the imperfect separation of data traffic and optical pump. The first impairment leads to the power budget constraint on the data traffic, and the second impairment results in degraded signal-to-noise ratio of the data traffic. Based on these, the general framework of QPON has been established in the stage 1 of the project, and the major limitations have been analyzed and better understood. The framework will be the basis for the next stage of this project, where we will investigate the power budget and signal-to-noise ratio in the end-to-end data transmission (i.e., involving multiple network nodes from the central office to the end user).
2. Framework of remote powering
One unique advantage of the proposed QPON is the capability of being powered remotely to eliminate the requirement of local power supply. The remote powering is realized using optical pump that is transmitted from the neighbouring nodes with local power supply or from the central office. The optical pump is transmitted together with the data traffic. Due to the relatively high power levels of optical pump, it is preferable to transmit it for a shorter distance together with the data traffic. Therefore, in our framework, we will use the nearest node with power supply to provide the optical pump.
Wavelength Band | 850 nm | 1310 nm | 1550 nm |
O-E Conversion Efficiency | 0.5-0.6 A/W | 0.8-0.9 A/W | 1.0 A/W |
Fibre attenuation | 1.8-1.9 dB/km | 0.4-0.5 dB/km | 0.2-0.3 dB/km |
When the optical pump arrives at the QPON without local power supply, it is first separated from the data traffic. Since the optical pump is typically much stronger than the data traffic, the leakage due to imperfect separation can be detrimental. One way to solve this issue is using different wavelength bands for the data traffic and the optical pump. Normally the 1550 nm wavelength band is used for the data traffic due to the low loss property through the optical fibre. Therefore, the 850 nm and 1310 nm wavelength bands can be promising options for the optical pump for remote powering, considering the possible leakage to the data traffic. In this project, we have studied these options in details theoretically, considering the optical fibre propagation attenuation and the optical-to-electrical (OE) conversion efficiency. Typical values of commercially available components in these wavelength bands are summarized in Table 1. For the 850 nm optical pump option, although the leakage to the data traffic is the lowest, it suffers from the highest fibre attenuation and the lowest OE conversion efficiency. On the other hand, the 1550 nm optical pump option has the lowest fibre attenuation and the highest OE conversion efficiency, it results in the highest leakage to the data traffic. Therefore, the 1310 nm wavelength band is a balanced option for the remote powering of QPON.
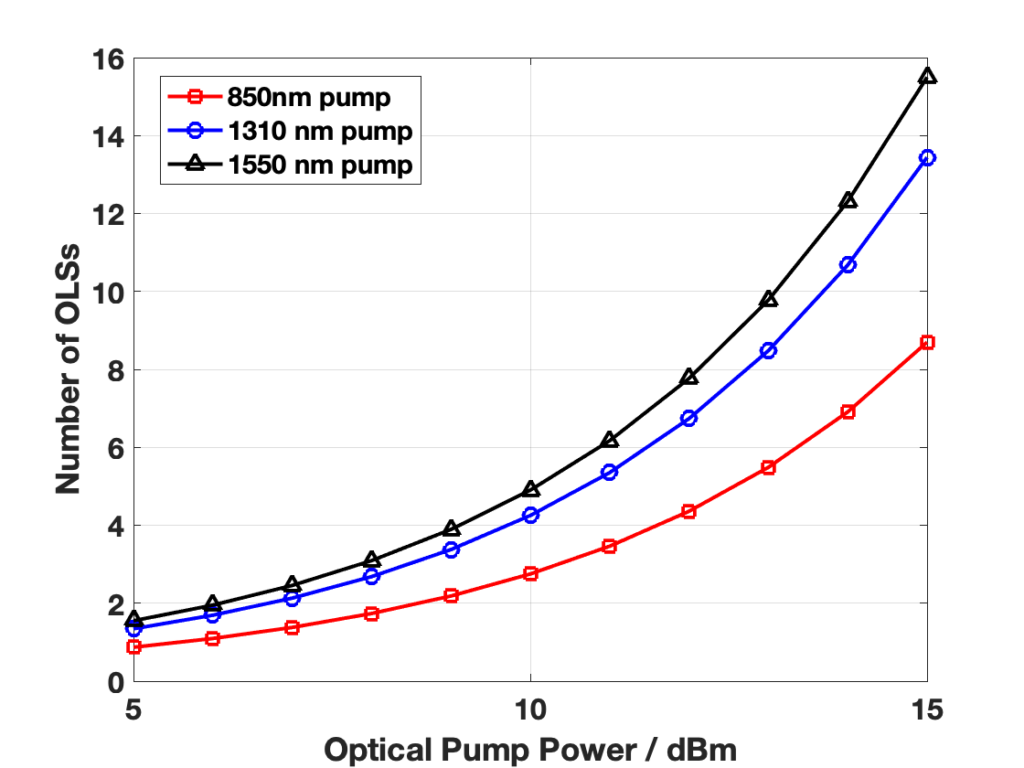
In QPON, the optical pump signal is converted to the electrical domain to provide the energy needed for the network node operation. The major power consumption components inside the QPON are the OLSs. In the stage 1 of this project, in order to understand the scalability of the QPON under remote powering, we have investigated the number of OLSs that can be powered simultaneously. The results are summarized in Figure 3. 2*2 OLSs are considered, since they can be considered as the universal building block for higher dimension OLSs or QPON. Considering practical limitations, we set the maximum optical pump power arriving at the QPON input at 10 dBm. The number of OLSs that can be power together is 2, 4 and 5 for 850 nm, 1310 nm and 1550 nm optical pumps, respectively. Considering the number of OLSs needed in each QPON, 1*2*2*2 QPONs can be remotely powered (i.e., 1 input port, 2 data traffic wavelengths, 2 signal parts divided, and 2 output ports).
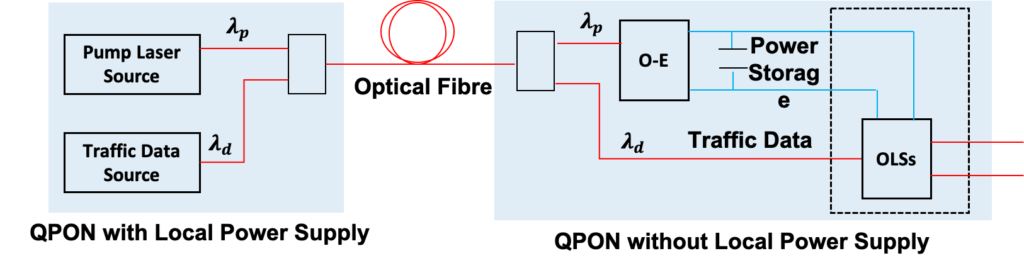
The remote powering scheme above is the direct remote powering, where the power converted from the received optical pump is used for powering the QPON immediately. However, to support larger QPON that is also needed in practice, we also study the indirect remote powering scheme, which is shown in Figure 4. Here, power storage components are used to store the power from optical pump when power consumption is not needed. This scheme is especially useful in the QPON due to the quasi-passive property, where power consumption is not needed in most cases (only consumed during re-routing or connection reconfiguration). Through this way, a larger number of OLSs can be powered remotely. There are multiple options available for the power storage component. Here we select to use the supercapacitor. This is because compared with other power storage components, such as the batteries, supercapacitors can accept and deliver charges more rapidly, support a larger number of charging and discharging cycles, and have fewer safety issues.
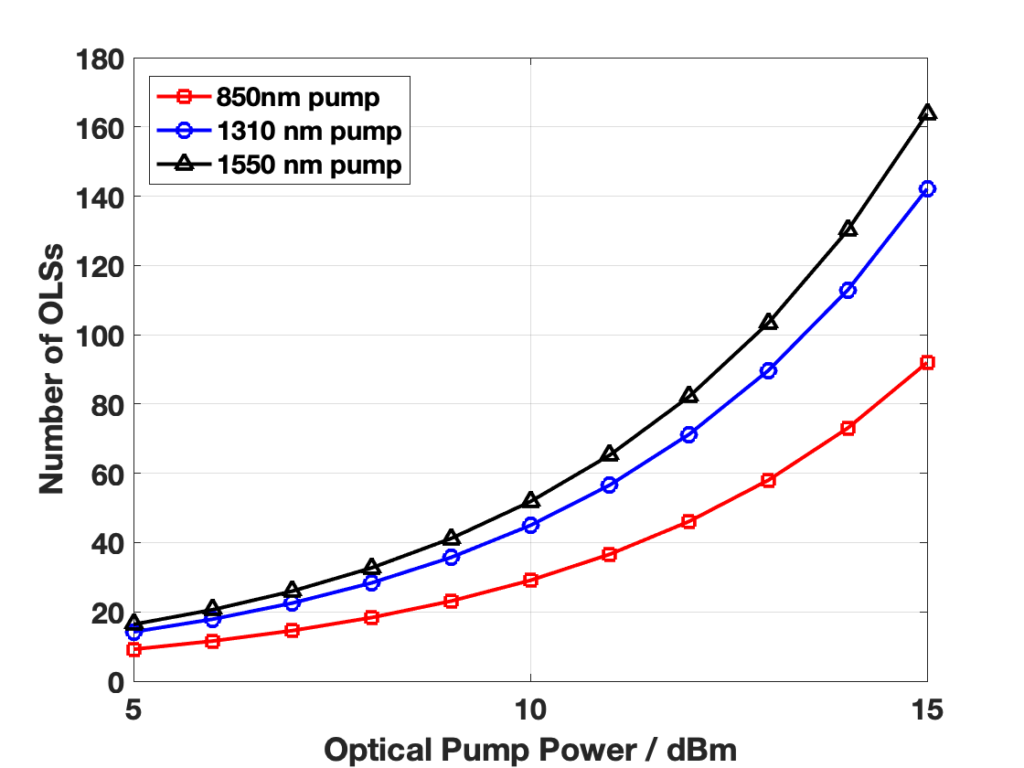
In order to better understand the capability of the remote powering scheme, especially the capability to support scalable QPON that is also needed in practice, we use the framework established above to theoretically study the number of OLSs that can be supported simultaneously. The results are shown in Figure 5. The parameters of the supercapacitor are selected according to the commercially available components, and we assume that the QPON needs to be reconfigured every 1s. Different optical pump wavelengths are considered. It can be seen that a much larger number of OLSs can be supported. Considering a practical optical pump power of 10 dBm, more than 45 OLSs can be supported using the 1310 nm pump wavelength. This indirect remote powering scheme significantly improve the scalability of the QPON. Both remote powering schemes, namely the direct and the indirect remote powering, is transparent to the modulation format, as long as the leakage to the data channel is small. This is an advantage in reality as the data traffic transmitted in the access network can be flexible to satisfy different needs.
In summary, during the stage 1 of this project, we have established the framework of both QPON and remote powering schemes. Based on the framework, we have conducted theoretical studies on the capability and limitation of QPON. The scalability issue, efficiency, and modulation compatibility have also been studied. The outcomes of stage 1 form the foundation for the next 2 stages.
Stage 2
1. Remote powering and data transmission
The framework of QPON remote powering to provide high-speed Internet access to remote communities was established in the Stage 1 of this project. Two possible remote powering schemes have been studied, which are based on the direct remote powering and indirect remote powering, respectively. Regardless of the remote powering scheme used, strong optical pump is always needed to the QPON nodes without local power supply and from the QPON nodes with local power supply. Therefore, a strong optical pump propagates together with the data-carrying signal in the optical fibre. With the existence of relatively strong pump, the data channel may suffer from three types of nonlinearities: (1) self-phase modulation; (2) cross-phase modulation; and (3) four-wave mixing. Based on the corresponding theories, we can evaluate the impact of pump on the data channel.
In this project, we consider different pump wavelength bands, different pump power levels, different co-transmission distances, and different data modulation formats. Here we keep the data channel at 1560 nm (i.e., C-band that is widely used in optical networks). We consider the following modulation formats due to the wide use in access networks: on-off-keying (OOK), 4-pulse-amplitude-modulation (4-PAM), and 8-pulse-amplitude-modulation (8-PAM). Other more advanced modulation formats have been considered in research as well. However, due to the complexity, they are currently not widely used in access networks. In addition, we consider both 1310 nm and 1550 nm optical pump possibilities as listed in Table 1 of Stage 1. The 850 nm optical pump is not considered here due to the very high optical fibre transmission loss, which significantly limits the maximum possible distance between QPON nodes. The results are presented in the following sections.
2. Optical pump at 1310 nm
In this section, we study the use of 1310 nm optical pump in QPON either with or without local power supply. We conduct extensive studies to investigate the co-transmission of optical data signal and pump signal. We divide the studies according to the data modulation format (OOK, 4-PAM and 8-PAM).
2.1 OOK data modulation
We first consider 10 Gbps data modulated with the OOK format. The signal wavelength, as discussed in the previous section, is selected at 1560 nm. The signal power generated by the laser is fixed at 10 dBm (before signal modulation, where external modulation is used). Key parameters considered are summarized in Table 2.
Signal Wavelength | 1560 nm | Pump Wavelength | 1310 nm |
Signal laser power | 10 dBm | Pump launch power | 50 mW to 500 mW |
Signal data rate | 10 Gb/s | Optical fibre length | 10 km to 100 km |
MUX/DEMUX loss | 0.5 dB per unit | Fibre loss | 0.2 dB/km (1560 nm) 0.5 dB/km (1310 nm) |
We assume that there is no optical amplifiers in the optical fibre link between adjacent QPON nodes. We use this assumption since we are considering the rural areas with difficulty to access power supplies (optical amplifiers normally require local power supplies to operate). The received optical pump power, data signal power, and bit-error-rate (BER) performance of transmitted data are shown in Fig. 6.
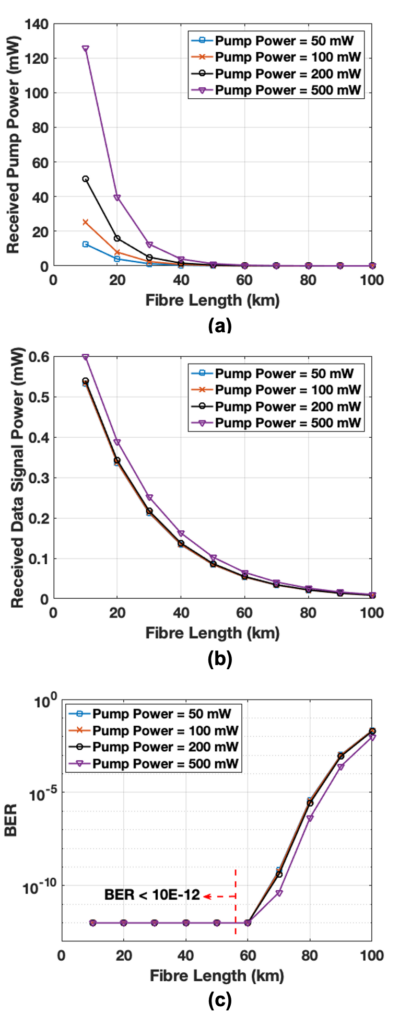
It can be seen from the results shown above that:
- The pump power decreases rapidly when the increase of fibre transmission distance when 1310 nm pump is used;
- The signal power decreases much more slowly compared with the pump power when the fibre transmission distance increases;
- The signal power is almost independent of the pump power. When the pump power increases to 500 mW, the signal power increases only slightly. This is mainly caused by the nonlinearity of the optical fibre;
- The BER performance is also almost independent of the pump power;
- For pump power of up to 200 mW, there is no noticeable impact of fibre nonlinearity in the system (i.e., signal power and BER keep constant when the pump power changes from 50 mW to 200 mW);
- Considering the remote powering requirement (at least 20 mW considering the power requirement of OLS), the fibre transmission length is limited to about 10-20 km when 1310 nm pump is used. In this case, the BER performance of the transmitted data satisfies the quality-of-service requirement. However, such fibre distance is too short for practical applications.
2.2 4-PAM data modulation
We now consider 10 Gbps data modulated with the 4-PAM format. The signal wavelength is kept at 1560 nm, and the laser output power is fixed at 10 dBm (before signal modulation, where external modulation is used). When 4-PAM is used, the symbol rate is reduced to 5 GBaud/s due to the better spectral efficiency (2 bits/s/Hz). Other key parameters considered are the same as those summarized in Table 2. The results are shown in Fig. 7.
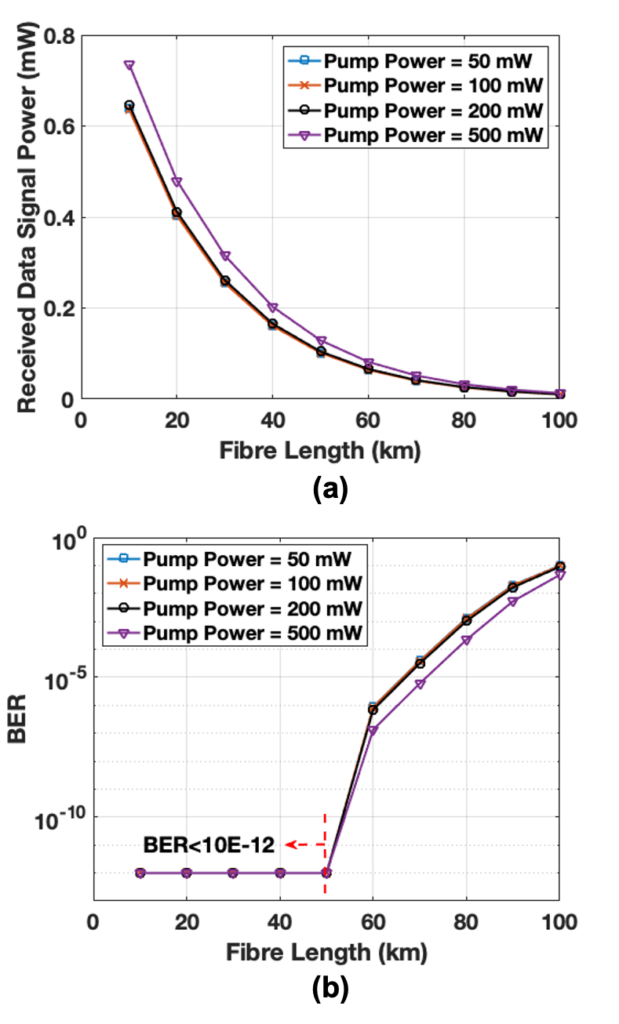
The results on received pump power are not shown, since they are the same as shown in Fig. 6(a). The results on the received signal power and BER after fibre transmissions follow that same trend as the case when OOK is used for data modulation. On the other hand, comparing the BER performances shown in Fig. 6 and Fig. 7, it is clear that the BER performance is worse when 4-PAM is used. This is mainly because that the current system configuration is power limited (instead of dispersion limited). When 4-PAM is used, due to the larger number of signal levels (4 v.s. 2 in OOK), a higher signal-to-noise ratio (SNR) is required to achieve the same level of BER. Therefore, the 4-PAM system performs worse.
2.3 8-PAM data modulation
We also consider 10 Gbps data modulated with the 8-PAM format. The signal wavelength is still kept at 1560 nm, and the laser output power is fixed at 10 dBm (before signal modulation, where external modulation is used). When 8-PAM is used, the symbol rate is reduced to 3.33 GBaud/s due to the better spectral efficiency (3 bits/s/Hz). Other key parameters considered are the same as those summarized in Table 2. The results are shown in Fig. 8.
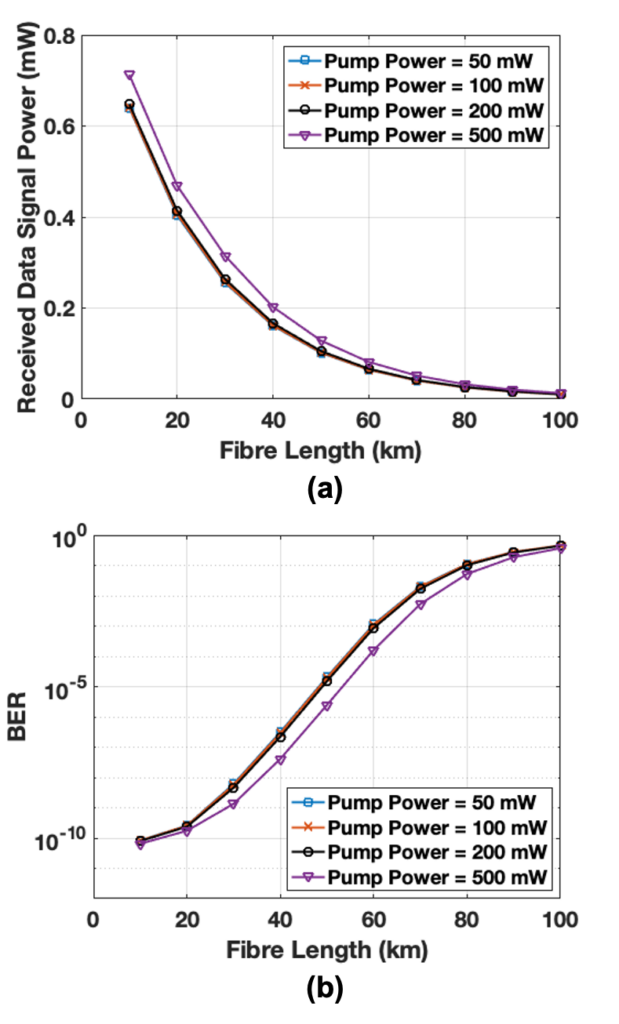
Similar with the 4-PAM case, the received pump power is not shown, since it is the same as the OOK case. The BER performance when 8-PAM is used is further worse compared with the 4-PAM case, due to the even larger number of signal levels to be determined. Hence, larger signal-to-noise ration and higher signal power are needed to achieve the same BER.
2.4 Impact of optical pump on the optical data signal
To further investigate the impact of optical pump on the optical data signal, we simulate the data signal spectrum (optical spectrum) before and after fibre transmission with different optical pump powers. In the study, we keep the signal wavelength at 1560 nm, the pump wavelength at 1310 nm, data modulation format at 4-PAM, fibre transmission distance at 50 km, and signal data rate at 10 Gb/s. Tow pump power levels are considered: 100 mW or 500 mW, corresponding to a relatively moderate level and a high level of remote power delivery, respectively. The optical spectrum results are shown in Fig. 9.
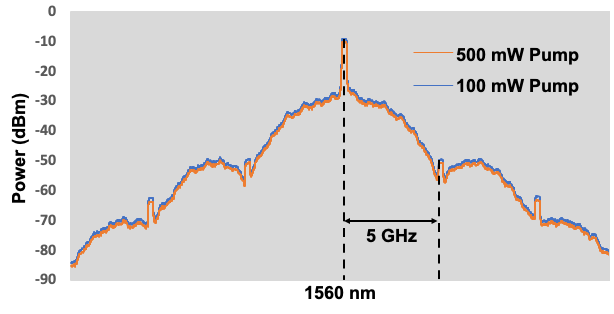
A few key points can be seen from the results shown in the figure:
- The optical pump co-transmission in the fibre has some impact on the data signal, whilst the impact is relatively weak when 1310 nm is used for optical remote power delivery. This is mainly due to the large wavelength difference between the data signal and the pump, which leads to relatively weak nonlinear effects.
- When the pump becomes stronger, the signal power after fibre transmission is slightly reduced, confirming the nonlinear effect. The major nonlinear effect is the four-wave-mixing (FWM), where new idler signal with different wavelength is generated. This process reduces the power of the data signal.
2.5 Conclusions – 1310 nm optical pump
In this part (Section 2 of Stage 2), we have studied one possible optical pump wavelength band, 1310 nm, as the optical pump to provide remote power delivery to QPON without local power supplies or from QPON with local power supplies. The 1310 nm pump is co-transmitted together with the optical data signal at 1560 nm in the standard single-mode optical fibre. We have focused on the point-to-point scenario directly between QPON nodes, and the findings are valid for networked QPON nodes as well.
From the studies and results above, it has been found that the use of 1310 nm band as the optical pump has the following key advantages:
- The optical pump has minimal impact on the optical data signal, and hence, the BER performance of the data signal is not noticeably degraded;
- 1310 nm pump sources with high output power are commercially available with relatively low costs.
However, the use of 1310 nm band as the optical pump also has the important and fundamental limitation of:
- The optical fibre propagation loss is too high at the 1310 nm band. Hence, the optical pump power decreases rapidly with the increase of signal transmission distance.
- Due to this, the power efficiency of 1310 nm based remote powering is highly limited (i.e., a large portion of power is wasted).
- The high propagation loss at the 1310 nm wavelength band also limits the maximum distance that is possible between adjacent QPONs. This also leads to further constrains in terms of the network topology.
3. Optical pump at 1550 nm
In addition to the 1310 nm band, in this section we study the use of 1550 nm optical pump for the remote powering of QPON. Similar with the previous section, we conduct extensive simulations to investigate the co-transmission of optical data signal and pump signal. We also divide the studies according to the data modulation format (OOK, 4-PAM and 8-PAM). In this section, we first fix the data rate at 10 Gb/s and the signal wavelength at 1560 nm. The pump wavelength is selected at 1550 nm. The signal power generated by the laser is fixed at 10 dBm (before signal modulation, where external modulation is used). Key parameters considered are summarized in Table 3.
Signal Wavelength | 1560 nm | Pump Wavelength | 1550 nm |
Signal laser power | 10 dBm | Pump launch power | 50 mW to 500 mW |
Signal data rate | 10 Gb/s | Optical fibre length | 10 km to 100 km |
MUX/DEMUX loss | 0.5 dB per unit | Fibre loss | 0.2 dB/km (1560 nm) |
3.1 OOK data modulation
We first consider the OOK format. The results are shown in Fig. 10.
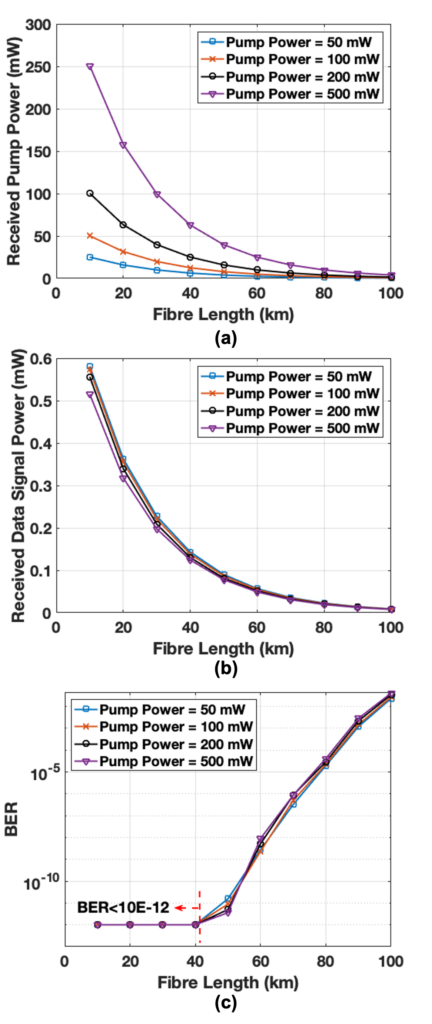
It can be seen from the results that compared with the 1310 nm pump case, the received pump power decreases much slower when the fibre transmission distance increases. This is mainly due to the lower fibre propagation loss at the 1550 nm band. This is an important and desirable feature when considering the remote power delivery to QPON without local power supplies. Considering the remote powering requirement (at least 20 mW considering the power requirement of OLS), the fibre transmission length can be up to 70 km when 500 mW pump is used. Even for 100 mW pump (i.e., 20 dBm), up to 40 km can be achieved.
However, when the 1550 nm pump is used, the impact of the optical pump on the performance of the data signal becomes stronger. From Fig. 10(b), it is clear that when the optical pump power level increases, the available data signal power decreases with the same optical fibre transmission distance. This is mainly caused by the four-wave-mixing effect, where extra idler wavelength is generated that consumes the data signal power.
The co-transmission of 1550 nm optical pump also affects the BER performance. Compared with the results shown in Fig. 6(c), which is the 1310 nm pump case, when the transmission distance is short, the impact of optical pump is not obvious. However, when the transmission distance increases, the BER degrades due to the impact of fibre nonlinearity. Fortunately, for the 10 Gb/s case and when considering the remote power requirement discussed above, the BER is well below the forward-error-correction (FEC) limit, and error-free transmission of the data signal can be achieved.
3.2 4-PAM and 8-PAM data modulation formats
We also consider the more spectral efficient 4-PAM and 8-PAM formats. The results are shown in Fig. 11 and Fig. 12, respectively. It can be seen from the results that compared with the OOK case, the impact of optical pump on the performance of data signal becomes more obvious when the higher-order modulation is used, where the received signal decreases more rapidly and the BER degrades more rapidly with the increase of optical fibre transmission distance.
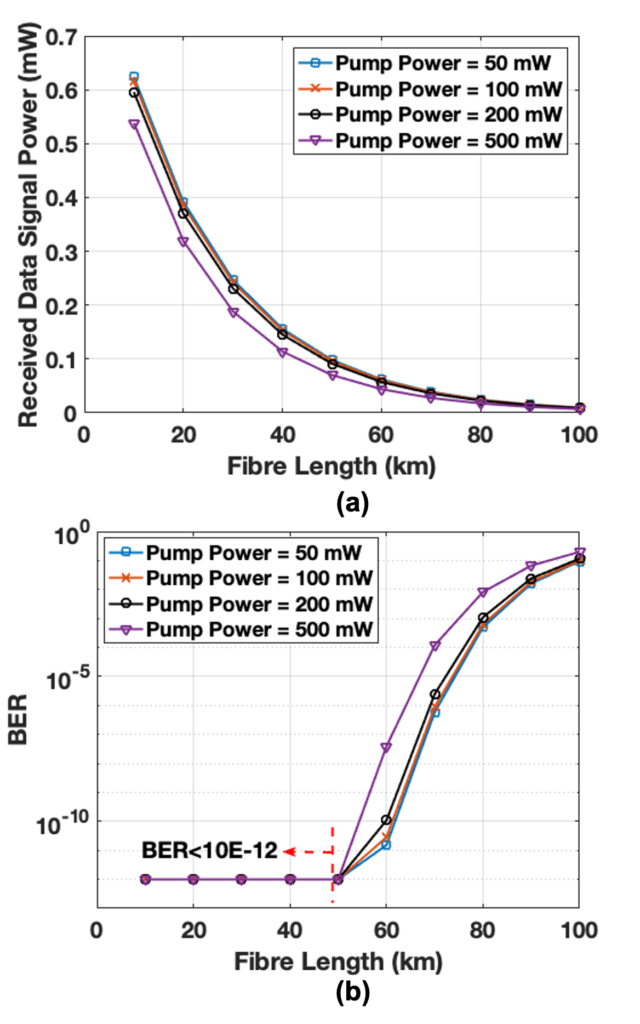
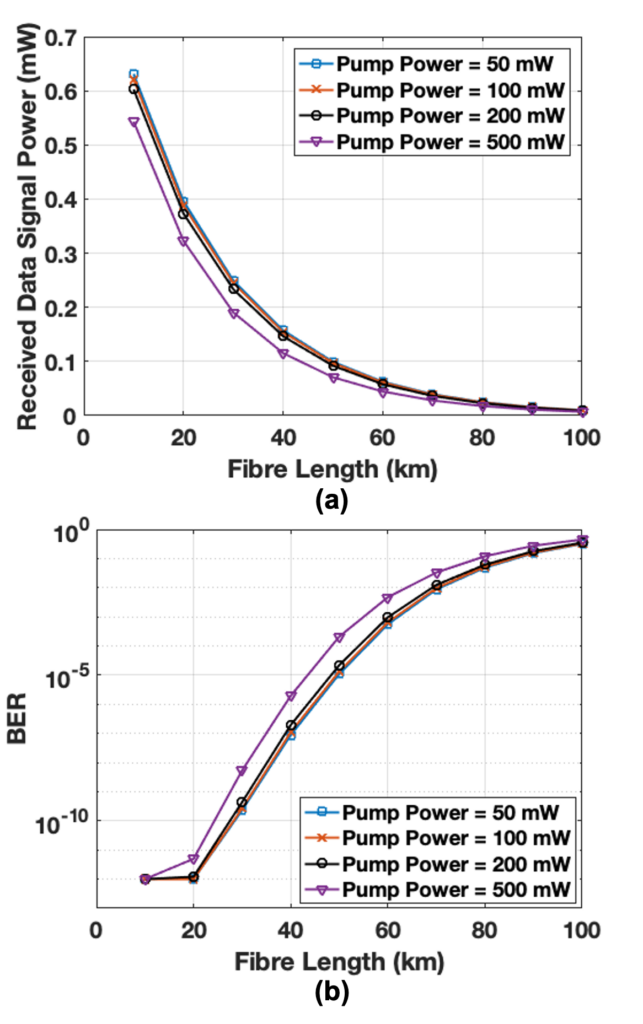
3.3 Conclusions – 1550 nm optical pump
In this section, we have studied the other possible optical pump wavelength band of 1550 nm. Compared with the 1310 nm optical pump scheme:
- The 1550 nm pump scheme provides substantially improved remote power delivery capability, where more power can be provided to the QPON without local power supply and the fibre transmission distance can be longer between QPON nodes.
- On the other hand, the use of 1550 nm optical pump scheme has larger impact on the quality of data-carrying optical signal transmission, due to the stronger nonlinear effects between the pump and the signal. This leads to worse BER performance and slightly lower received optical signal power.
- In addition, compared with the 1310 nm, whilst the high power pump lasers at 1550 nm are also commercially available, the cost is higher.
Considering both remote power and data transmission aspects, we reach the conclusion that the 1550 nm optical pump scheme is the better option. This is because that it provides reasonably good data signal transmission quality, and at the same time, provides the remote power delivery capability over a reasonable distance. Due to this, in the subsequent investigations, we will focus on the 1550 nm remote power scheme.
Regarding the data modulation format, the results in this section indicate that the simple OOK provides the best BER performance. This is mainly due to the lower signal-to-noise required by OOK to achieve the same BER. However, the OOK has low spectral efficiency that limits the achievable bit rate. In addition, only 10 Gb/s data is considered in this investigation, which is relatively low speed. When the data rate increases, the impact of chromatic dispersion becomes stronger, and the OOK is more vulnerable to further BER degradations. Therefore, the modulation format needs to be further analyzed.
4. Data rate and modulation format investigations
In the previous sections (Section 2 and Section 3, Stage 2), 10 Gb/s data transmission is considered. For practical considerations, such data rate can be considered as relatively low speed, especially given the relatively long distance between QPON nodes. Therefore, in this section we further investigate the impact of data rate on the data transmission performance. As discussed in the previous section, here we consider the use of 1550 nm optical pump scheme in the following investigations.
4.1 OOK format
In this section, we look at the use of OOK modulation for data transmission at different data rates. Based on the requirement of getting sufficient power delivered to the QPON without local power supply, here we consider the optical pump of 200 mW and 500 mW, and the optical fibre transmission distance of 50 km and 60 km. We also consider the cases with and without fibre dispersion compensation. The key parameters used in the following studies are summarized in Table 4.
Signal Wavelength | 1560 nm | Pump Wavelength | 1550 nm |
Signal laser power | 10 dBm | Pump launch power | 200 mW or 500 mW |
Signal data rate | 20 Gb/s, 40 Gb/s or 50 Gb/s | Optical fibre length | 50 km or 60 km |
MUX/DEMUX loss | 0.5 dB per unit | Fibre loss | 0.2 dB/km |
The data transmission results are shown in Fig. 13.
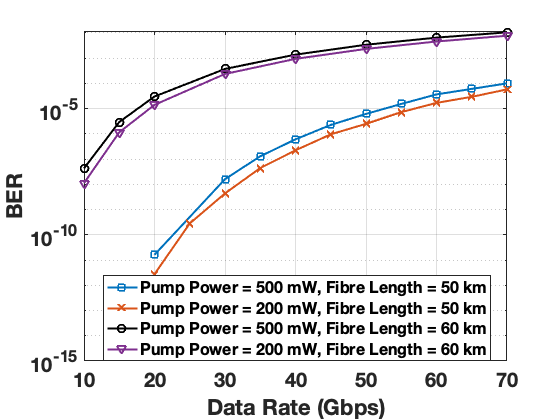
From the results, it can be seen that:
- When the data rate increases, the BER performance degrades, which is consistent with the expectation, due to the broader data bandwidth needed.
- When the fibre distance between adjacent QPON nodes increases, for the same data rate and the same optical pump selection, the BER decreases. This is mainly caused by the more severe nonlinearity in the system, as the longer fibre distance provides a longer path for nonlinear interaction.
- In addition, for the same data rate and the same fibre distance between adjacent QPON nodes, the BER performance becomes worse when the optical pump power increases. This is also caused by the more severe nonlinearity.
4.2 PAM-4 and PAM-8 modulation formats
We also investigate the use of PAM-4 and PAM-8 modulation formats under different data rates, as these modulation formats are more spectral efficient compared with the OOK format. The parameters used in the study are the same as the OOK case (i.e., shown in Table 4). The results are shown in Fig. 14 and Fig. 15, respectively.
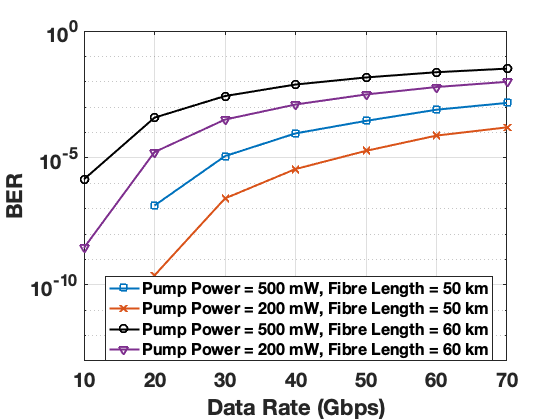
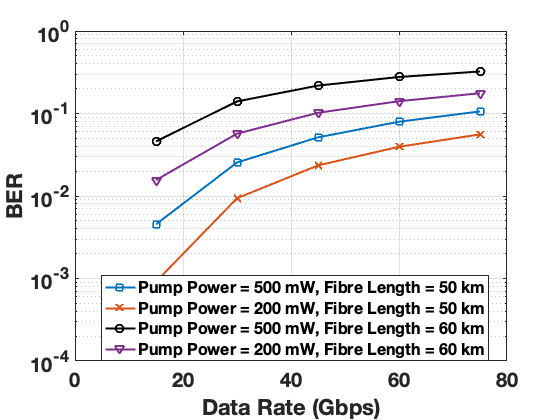
Compared with the OOK case, the impact of nonlinearity becomes more obvious when PAM-4 or PAM-8 is used. This can be seen from the larger performance difference under different optical pump powers, or with different fibre transmission distance between QPON nodes. In addition, the BER performance of PAM-4 and PAM-8 is also worse compared with the OOK. This is because that a larger number of signal power levels need to be determined in PAM-4 or PAM-8, which requires higher signal-to-noise ratio and larger received optical power to reach the same performance.
Although the PAM-4 and PAM-8 have the disadvantages discussed above, it also has the important advantage of occupying narrower bandwidth. This is especially important in advanced optical networks where the wavelength-division-multiplexing (WDM) is widely deployed. In WDM, the channel spacing between adjacent data-carrying signal channels is limited, and hence, more spectral efficient modulation format becomes more important as it can enable higher communication data rate. For example, under the 50 GHz standard WDM channel spacing (defined by ITU-T), the date rate of OOK will be limited to about 40 Gb/s when considering the guard band required between channels. On the other hand, 80 Gb/s can be provided by using PAM-4 and 120 Gb/s can be realized via PAM-8. However, as shown in Fig. 15, the PAM-8 BER performance is much worse than the OOK and the PAM-4 cases. Therefore, considering all the aspects discussed above, the PAM-4 format is the best option for the data transmission together with the remote powering technology.
Stage 3
Due to the impact of COVID and the resulting lockdown, the lab access becomes highly limited. In addition, one key piece of equipment, which is the high power lasers, is unexpectedly out of stock and the re-stock has taken much longer than previously expected (over 12 months delay). Hence, after seeking approval, we have made some changes to the stage 3 of this project. These include: reduce the amount of experimental investigation, investigate more advanced remote powering schemes, and add additional theoretical studies and numerical simulations to investigate networked QPONs (i.e., larger-scale and interconnected QPONs for more practical scenario). The key results and findings are summarized in this section.
1. Multiple optical pumps scheme:
In addition to the single pump possibility, there is another possibility of using multiple pumps. This significantly reduces the requirement on the pump light sources and at the same time, increases the power that is available at the remote quasi-passive optical node that does not have local power supply. Hence, in this section, we investigate the feasibility and different configurations of the multiple optical pumps scheme.
According to previous studies, it has been shown that for the optical pump, the 1550 nm wavelength band is better overall compared with the 1310 nm wavelength band. Therefore, in this part of study, we focus on the use of 1550 nm band for optical pump, and we optimize the performance of both the data signal and the optical pump.
Using the 1550 nm band for the optical pump, the multiple optical pumps principle can be implemented using two ways:
- Multiple optical pumps located at the same side of the data signal (i.e., wavelengths of all optical pumps longer or shorter than the wavelength of the data signal).
- Multiple optical pumps located at different sides of the data signal (i.e., some optical pumps have longer wavelengths compared with the wavelength of the data signal, and some optical pumps have shorter wavelengths compared with the wavelength of the data signal)
Here we investigate both possible configurations of the multiple optical pumps scheme. In the investigation, the key parameters used are listed in Table 5.
Signal Wavelength | 1560 nm | Pump Wavelengths | 1550 nm band with 0.8 nm spacing (100 GHz grid) |
Signal laser power | 10 dBm | Pump launch power | 200 mW or 500 mW per pump |
Signal data rate | 10 Gb/s up to 100 Gb/s | Optical fibre length | 50 km |
MUX/DEMUX loss | 0.5 dB per unit | Fibre loss | 0.2 dB/km |
1.1 Scenario 1: all optical pumps have longer or shorter wavelengths than the wavelength of the data signal
We first study the case where the optical pumps are located at the same side of the data signal. Based on ITU-T standard, the channel spacing between the optical pumps is selected at 0.8 nm (100 GHz). In the study, we fix the optical fibre length at 50 km and the modulation format at 4-PAM (according to the investigation above). We first investigate the use of 2 optical pumps and the impact on the BER performance of the data signal that is co-transmitted in the same optical fibre. The results are shown in Fig. 16. It can be seen from the results that when stronger optical pumps are utilized, the BER performance of the signal degrades slightly, and this is mainly due to the stronger nonlinearity during the fibre propagation. Compared with the results shown in Fig. 14 where a single optical pump is used, we can see that the BER performance here is slightly worse, as the total optical pump power becomes larger. However, although the BER performance degrades slightly, it should be noted that the optical power that is delivered to the remote node without local power supply is increased significantly. Hence, the multiple optical pumps scheme is a feasible solution when the power requirement of the remote node is relatively high (e.g., the remote node to be remotely powered has a large dimension).
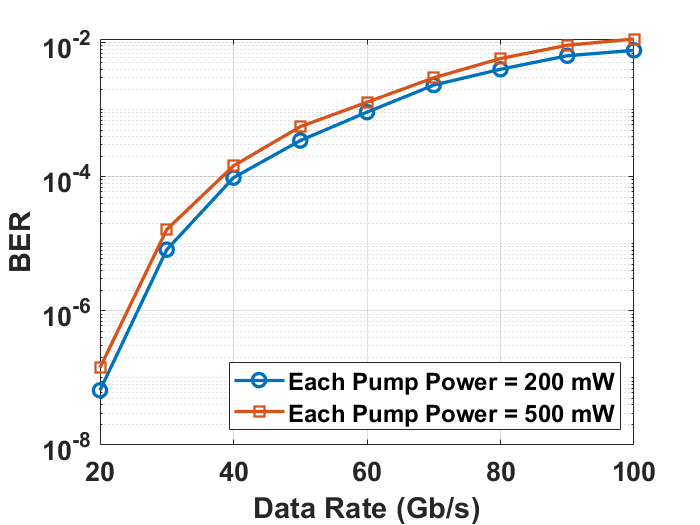
The results shown in Fig. 16 demonstrates the feasibility and benefits of using the multiple optical pumps scheme. Now we further investigate the use of different numbers of optical pumps. In this study, we fix the data rate at 40 Gb/s with PAM-4 format, and we also fix the fibre transmission distance to 50 km. The number of optical pumps ranges from 2 to 7, considering the practical cost and space limitations of the remote node with local power supply. The impact on both signal data and total optical power available at the remote node without local power supply is considered. The results are shown in Fig. 17 and Fig. 18, respectively.
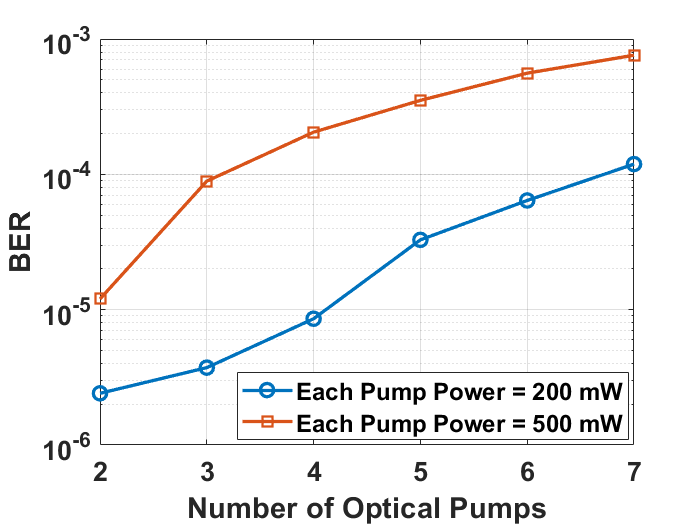
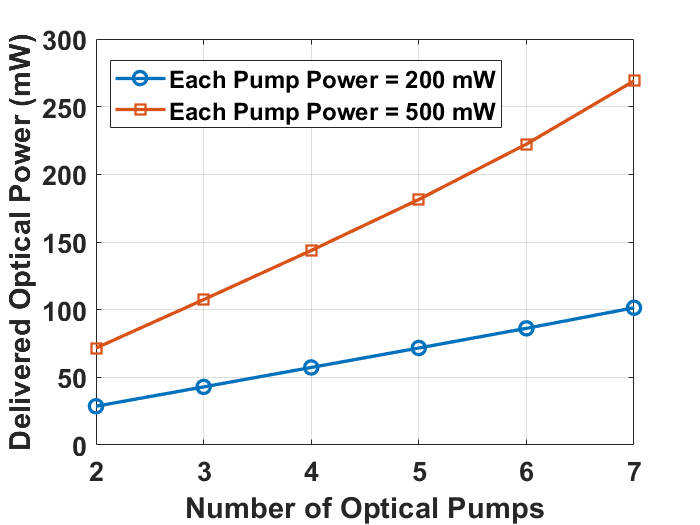
From the investigations above, it can be seen that:
- When there are a larger number of optical pumps used, the impact on the data signal co-transmitted in the optical fibre becomes more obvious: the BER degrades when a larger number of optical pumps are used.
- Compared with the multiple optical pumps scheme with 200 mW pumps, the impact of using 500 mW pumps is more significant.
- When considering the use of forward-error correction (FEC) in optical fibre communication systems and networks, up to 7 optical pumps (each with 500 mW) can be used for remote nodes without local power supply.
- The use of multiple optical pumps scheme can also significantly improve the power that can be delivered to the remote noted, where >250 mW delivery becomes possible. Therefore, based on the study in Section 2, up to 50 OLSs can be operated simultaneously to support QPON with relatively large dimension.
1.2 Scenario 2: optical pumps have both longer and shorter wavelengths than the wavelength of the data signal
In the previous section, the wavelengths of all optical pumps used are either longer or shorter than the data signal wavelength. Now we further consider a second possible scenario, where some optical pumps have wavelengths longer than the data signal, and the remaining optical pumps have wavelengths shorter than the data signal. Similar with the previous section, we start with the case where two optical pumps are employed.
The key parameters of the investigation are the same as those listed in Table 5. We also fix the optical fibre length at 50 km and the modulation format at 4-PAM (according to the investigation in section 3). The BER results of the optical data signal at different data rates are shown in Fig. 19. The general trend of the BER performance is similar with that shown in Fig. 16. However, compared with the case where both optical pumps are located at the same side of the data signal, here the BER performance difference between different optical pump power levels (i.e., 200 mW or 500 mW per optical pump) is smaller. In addition, also compared with the previous case, the BER performance becomes better in general using this configuration of the multiple optical pumps scheme.
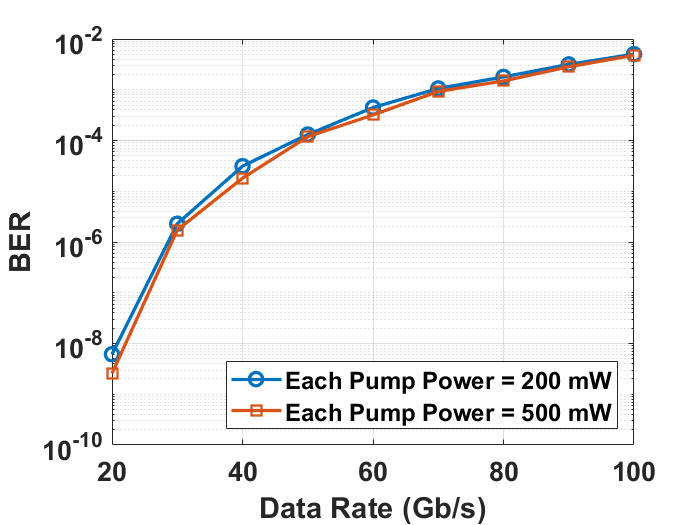
More investigations are also conducted by looking at the BER performance of the optical data signal and the optical power delivered to the remote QPON node when the number of optical pumps is change between 2 and 7. These optical pumps are located at different sides of the data signal in terms of the wavelength. Similar with the scenario 1, here we also fix the data rate at 40 Gb/s with PAM-4 format, and we also fix the fibre transmission distance to 50 km. The results are shown in Fig. 20 and Fig. 21.
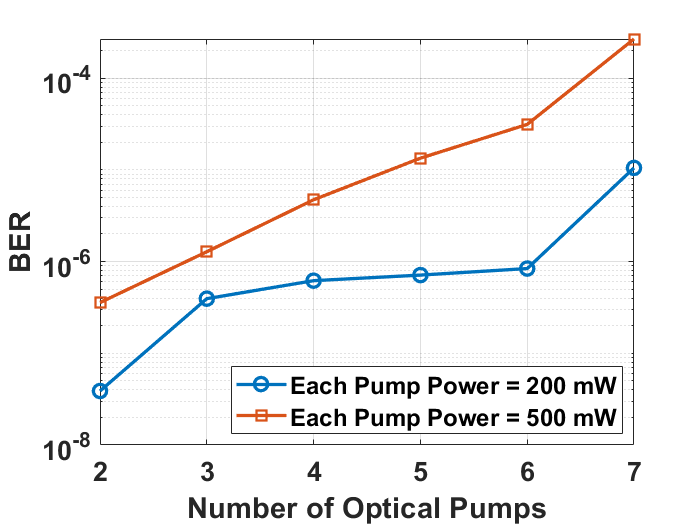
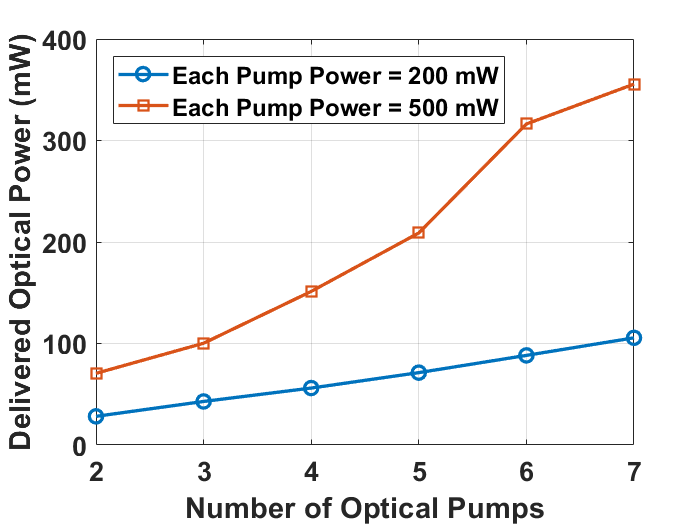
Compared with the previous multiple optical pumps configuration, it can be seen that this configuration leads to better performance, in terms of both the BER performance of the data signal as well as the optical power that can be delivered to the remote node. More specifically, with the same number of optical pump sources and the same power level from each optical pump source, the actual optical power that can be delivered to the remote node without local power supply can be increased by over 31.97%. Therefore, more OLSs can be powered in the QPON, and larger dimensional nodes can be supported. Compared with the single optical pump scheme, the multiple optical pumps scheme investigated here is more effective in the remote powering of QPON, especially when the power requirement of the QPON is relatively high.
2. QPON in optical networks
In the previous sections, we have investigated the QPON, including the general structure of QPON, the mathematical and theoretical model of QPON, and the use of QPON for data and power transmissions. We have studied different optical remote powering options (e.g., 1310 nm v.s. 1550 nm optical pumping), different data modulation formats and data rates, as well as the multiple optical pumps scheme. Results have shown that compared with the 1310 nm band, the 1550 nm band is a much better option for the remote powering of QPON without local power supply. In addition, compared with the single optical pump scheme, the use of multiple optical pumps also provides important advantages.
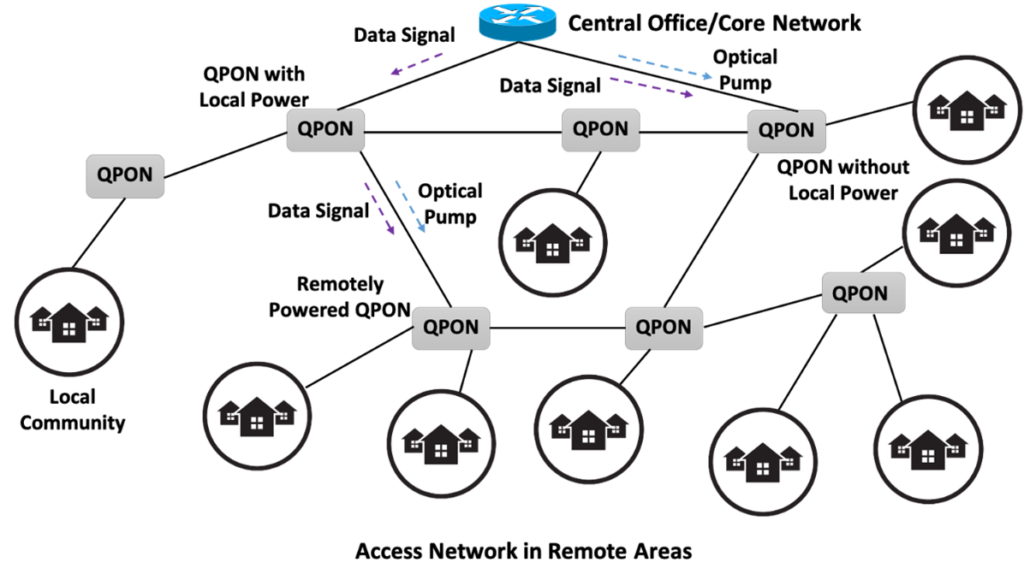
However, previous sections mainly focus on the single QPON. In this part, we will further investigate the use of QPON in optical networks. The optical network that we consider is shown in Fig. 22. Here we focus on the distribution of data signal from the core network to the access network in remote areas. Two types of QPON nodes exist in the network: (1) QPON with local power supply; and (2) QPON without local power supply. The general structure of these two nodes has been discussed in Fig. 1.
In the QPON-based optical network, the most important task is deciding the route of remote power delivery. Due to the propagation loss involved, it is always favourable to use the nearest QPON with local power supply. In this part, we conduct statistical simulations to gain insight on the power level that can be expected in practical optical access networks when the remote powering technique studies in this project is utilized.
In the statistical study, the overall area considered is 250 km × 250 km, and QPON nodes are distributed inside this area. We assume that there are 100 QPON nodes and there is a direct optical fibre link between neighbouring nodes. Each QPON node is equipped with Npump optical pump sources, and Npump can range from 1 to 7. The output power from each pump source is between 100 mW and 500 mW. For the optical pumps, the 1550 nm band is used. We assume that 50% of the QPON nodes have local power supply and the remaining 50% of nodes require remote powering. We conduct the simulations for 500 times and the statistical results are shown in Fig. 23. It can be seen that under the current setting, there is a high probability that up to 400 mW can be delivered to the QPON nodes without local power supply. However, there is also a good chance that <50 mW optical power is delivered, which means that the network may not be operated according to the requirement.
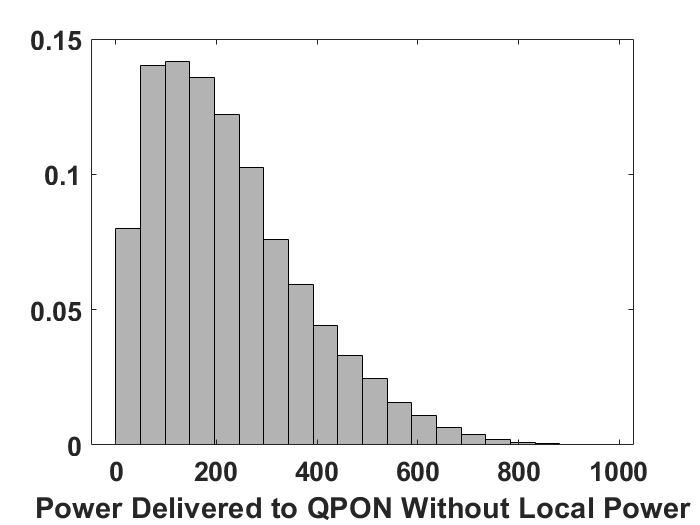
The possibility of getting the required amount of power delivered to the QPON nodes without local power supply can be improved by three major ways: (1) using more nodes; (2) increasing the power provided by each optical pump source; and (3) increasing the number of pump sources. We also verify this using extensive numerical simulations.
Firstly, we increase the number of nodes and investigate the impact on the power delivered. We keep the setting that 50% of the nodes have access to local power supply. The results are shown in Fig. 24 – Fig. 26, which corresponds to 120 nodes, 150 nodes and 180 nodes in this area, respectively. It is clear that when there are more nodes available in this area, the possibility that a higher power can be delivered to the QPON nodes without local power supply increases. This is because the effective distance between nodes now become shorter, and hence, the propagation loss of optical pumps is reduced and more power can be delivered.
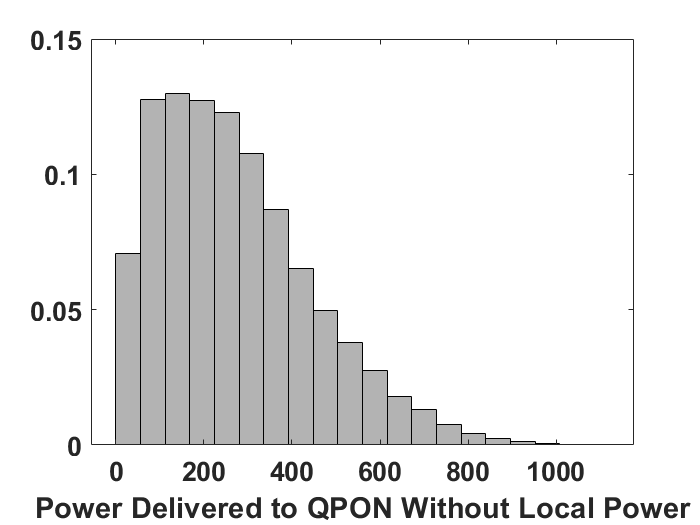
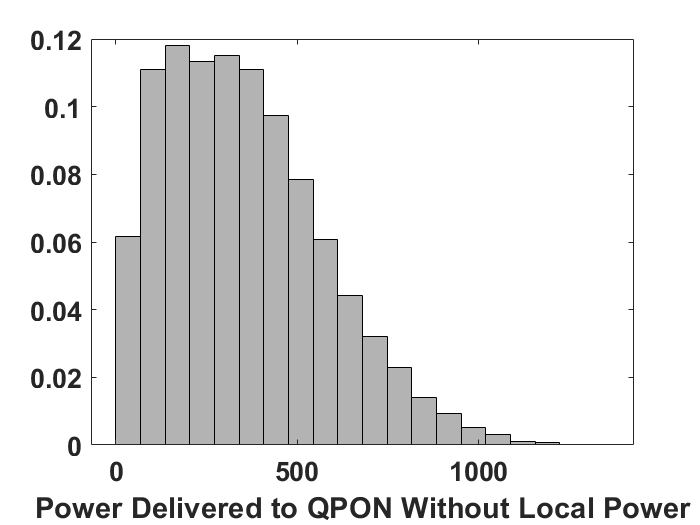
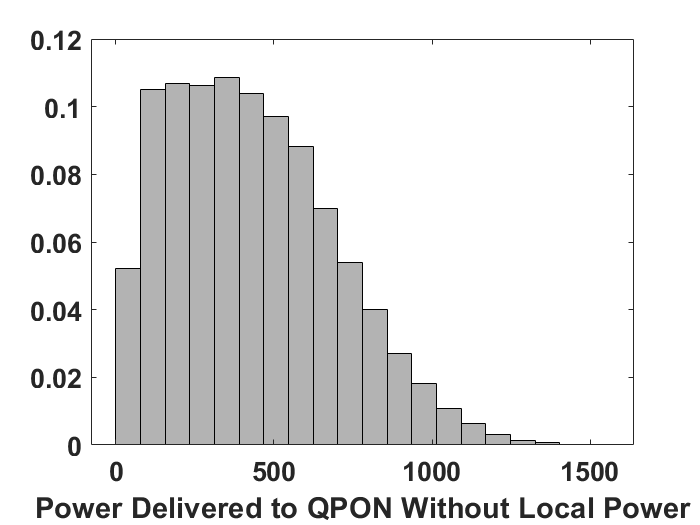
Secondly, we study the impact of the number of pump sources available in the QPON nodes with power supply. In this part, we keep the number of nodes at 100, and we maintain the setting that 50% of all nodes have local power supply access. The number of pump sources available now becomes 2-10 sources or 4-10 sources, and the results are shown in Fig. 27 and Fig. 28, respectively. It is clear that the number of pump sources available has substantial impact on the network remote powering performance. In particular, when a larger number of optical pump sources are available, the possibility that the power delivered to the QPON nodes without local power supply is very low (<40 mW) reduces significantly to almost 0%. This can be of great importance in practical applications, as in this case, at least small-scale network nodes can be supported in remote areas.
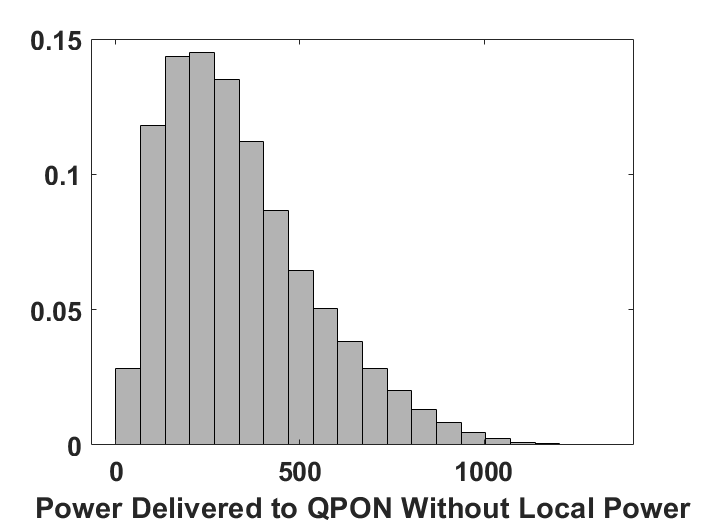
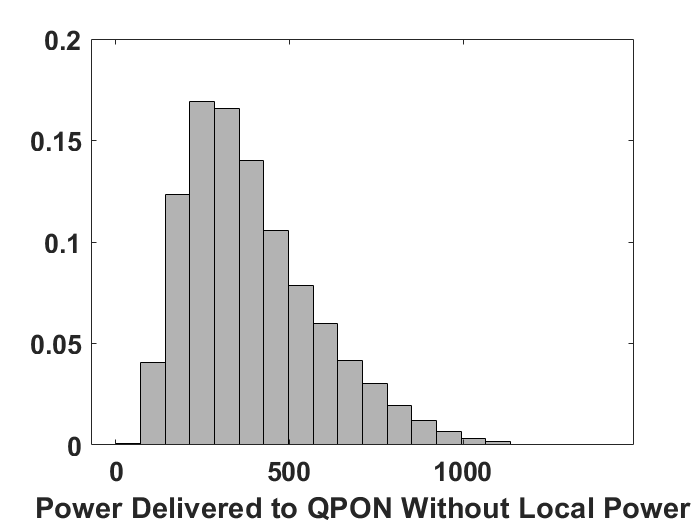
Finally, we study the impact of the power level from each optical pump source. Here, we keep the number of nodes at 100, we maintain the setting that 50% of all nodes have local power supply access, and the number of pump sources available is fixed at 1-7 sources. The power from each optical pump source ranges between 50-450 mW or 200-500 mW. The results are shown in Fig. 29 and Fig. 30.
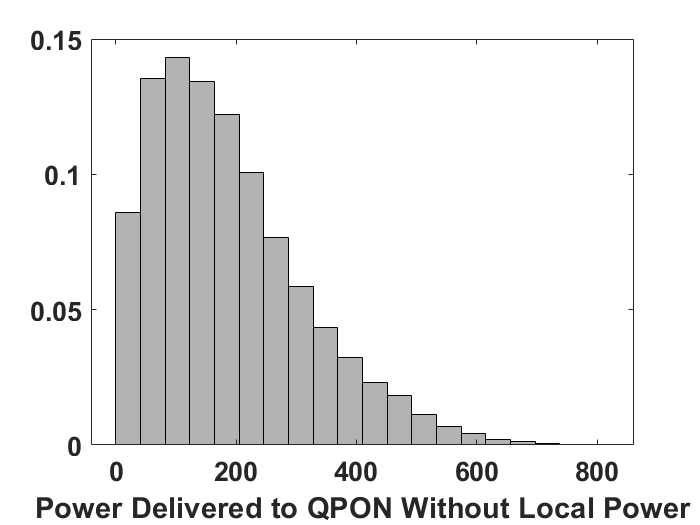
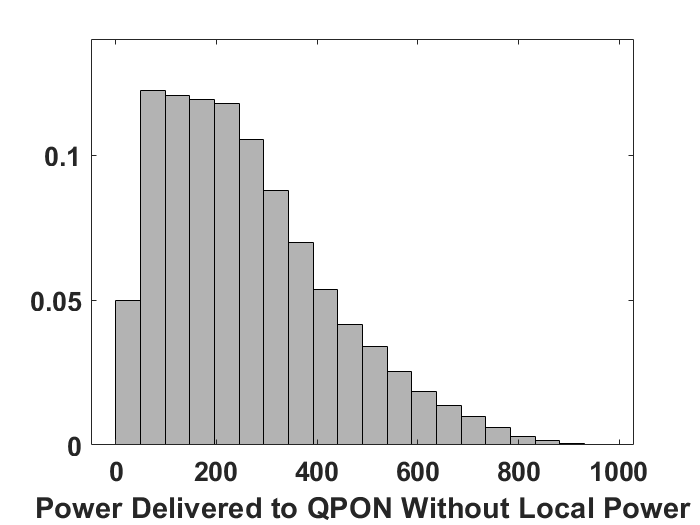
Based on the investigations in this section, it is clear that by increasing the number of optical pump sources, increasing the power transmitted from each optical pump source, and increasing the number of nodes, the remote powering technique proposed and demonstrated in this project can become more effective. Through this way, network nodes without local power supply can be enabled, which provides a promising solution to the delivery of high-speed network connections to remote areas.
Conclusions
In this project, we have established the framework of QPON for delivering high-speed network connections to remote areas. Two types of QPONs have been proposed and studies: the QPON without local power supply and the QPON with local power supply. In addition, we have established the framework for the remote powering technology, including both direct remote powering and indirect remote powering. The remote power technology has been investigated in details via both theoretical studies and comprehensive numerical simulations. The impact of strong optical pump on the data-carrying signal has been investigated in detail. More specifically, we have studied the selection of optimum optical pump wavelength and power, and the optimum modulation format. In addition, we have proposed and studied the multiple optical pumps scheme to further increase the amount of power that can be delivered to QPON without local power supply. Compared with the single optical power configuration, the use of the multiple optical pumps scheme has been shown to significantly increase the power available to power remote network nodes. Furthermore, based on the QPON proposed and studied, we have also conducted larger-scale network investigations when the QPONs are used. Large amounts of simulations have been carried out and the statistical property of the performance has been investigated. Results have shown that by optimising the density of network nodes and the optical pump sources used, QPONs without local power supply can be enabled with stable performance, which provides a promising solution to the delivery of high-speed network connections to remote areas.
Project Evaluation
The project so far has achieved the original objectives in stage 1 and stage 2, where the framework of QPON and remote powering has been established, the capability and limitation have been theoretically analyzed and different remote powering schemes have been studied comprehensively. These outcomes have enabled us to better understand remote powering in optical access networks to provide high-speed and reliable Internet to remote areas. The results have shown that both QPONs with and without local power supply are feasible and can be deployed according to practical scenarios. The results have also shown that the indirect remote powering scheme makes the QPON without local power supply scalable. In addition, the results have shown that the 1550 nm remote powering scheme provide more balanced performance when considering the remote power delivery, transmission distance and data communication aspectes simultaneously. The proposed solution in this project is promising in delivering high-speed and reliable network access to remote areas.
However, due to the impact of COVID-19, which results in lab access restrictions as well as the delay in obtaining the ordered component/equipment, the original objectives in stage 3 have been affected. To mitigate this impact, with approval we have adjusted the objective 3 to larger-scale network level investigations instead. With this change, the updated objective 3 of the project has been successfully achieved. In this stage, a new multiple optical pumps scheme to further increase the power that can be delivered to QPON without local power supply, and hence, it can enable larger dimensional QPON with more advanced functions. In addition, the use of QPON in optical networks has been investigated, where the statistical performance with QPON has been studied. Results have shown that the use of QPON and the remote powering technique can enable the wide availability of network nodes, and hence, the technique studies in this project is promising to enable delivering high-speed and stable network access to remote areas.
The day-to-day activities and study of the project have been conducted by a PhD student who started her candidature at the end of 2019. This project has provided excellent research training opportunity to the female PhD student. The support provided by the project also provides hands-on experience to the student. This project has encouraged “women in engineering” to support better gender equality. We also managed to involve another female student in this project (from the stage 2 of the project, the student is a Masters student doing the thesis project). The experience provided by the project to female students has enhanced the technical capability and showcased the concept of “women in engineering”, and we believe this can improve gender equality in the engineering discipline in the future.
There are three most important outputs of the project as follows:
- The establishment of the theoretical framework for QPON and remote powering in stage 1. This framework enables us to better explore the capabilities and limitations. More importantly, the framework is used as the foundation to optimize the QPON and remote powering scheme in the stage 2. In addition, preliminary simulations have also been completed. The simulations provide reliable feasibility study.
- The establishment and comprehensive study of possible remote powering schemes in stage 2. Two practical remote powering schemes using the 1310 nm and 1550 nm optical pump bands have been established and thoroughly studied. Results have shown that when considering the transmission distance, remote power delivery efficiency and capability, and data communications together, the 1550 nm remote powering provides the better solution. Extensive studies have also been conducted to understand the impacts of co-transmission of strong optical pump and data signal between QPON nodes. These outputs have verified the feasibility of the proposed remote powering technique, and have provided the optimized solution of using the 1550 nm optical pump.
- The establishment of the multiple optical pumps scheme in stage 3. Although the power can be remotely delivered to QPON without local power supply, due to the availability and cost of high power optical pump sources, the amount of power that can be delivered is relatively limited. This project successfully solves this limitation by the proposed multiple optical pumps scheme. Two possible configurations of this scheme have been investigated, and it has been found that by distributing the optical pump wavelengths to both sides of the data signal wavelength, the impact on the data transmitted can be reduced and the power delivered can be increased. This output provides a promising and practical way to provide sufficient power to large-dimensional QPON in remote areas, so that more advanced network functions can be supported by the remote network node.
- The key findings and outcomes of the project have been published via a scientific paper. The paper, entitled “Remote Power of Rural Network Nodes for Future Optical Networks,” has been presented at the IEEE International Conference on Electronics and Communication Engineering (2021) [17], and has been published and accessible widely through IEEE. The presentation in the conference has attracted considerable attention, and it has won the Best Paper/Presentation Award in the area of Mobile Communications Information Networks.
This project has further strengthened RMIT’s research capability in telecommunications, especially on high-speed optical communications and networks, by investigating an important practical problem through an innovative technical solution. The outcomes of this project has great potential in solving current limitations and enabling high-speed and reliable network connections to remote communities, to provide and realize significant social benefits beyond just academic research.
Indicators | Baseline | Project activities related to indicator | Outputs and outcomes | Status |
How do you measure project progress, linked to the your objectives and the information reported on the Implementation and Dissemination sections of this report. | Refers to the initial situation when the projects haven’t started yet, and the results and effects are not visible over the beneficiary population. | Refer to how the project has been advancing in achieving the indicator at the moment the report is presented. Please include dates. | We understand change is part of implementing a project. It is very important to document the decision making process behind changes that affect project implementation in relation with the proposal that was originally approved. | Indicate the dates when the activity was started. Is the activity ongoing or has been completed? If it has been completed add the completion dates. |
Establishment of the framework for QPON and remote powering | No such framework | Both QPON and remote powering schemes have been analyzed theoretically. Key components and steps have been considered and included. | Two QPON framework has been established: one for the QPON with local power supply and the other for the QPON without local power supply. Two remote powering schemes have been established: (1) direct remote powering scheme; and (2) indirect remote powering | This part starts from the beginning of this project as the stage 1. It has been completed in the stage 1 of the project. |
Optimization of the QPON structure and remote powering scheme | No optimization available | Based on the theoretical framework, optimize the QPON structure about the component need, and optimize the remote powering scheme for better efficiency. | Optimized QPON structure with low complexity, and optimized remote powering scheme with high efficiency. | This part starts from May 2020, and it is the stage 2 of project. This stage 2 has been completed so far. |
Investigations of larger-scale optical networks with the proposed QPON and remote-powering scheme | No prior network-level investigations existing | Conduct Conduct extensive larger-scale simulations to understand the expected performance of optical networks employing the QPON and remote powering technique | Larger-scale optical network with QPON and remote powering model has been established, and based on the model, extensive simulations have been conducted. Optimized remote powering schemes have been investigated to maximize the amount of power delivered. | This part starts from December 2020, and it is the revised stage 3 of project. This revised stage 3 has been completed so far. |
Gender Equality and Conclusion
In the initial proposal, for the gender equality and inclusion, we plan to involve two female students in this project: one PhD student for day-to-day research activity and another undergraduate student for practical project training. In the project, the female PhD student has conducted day-to-day research activities since the start of this project. This project not only provides support for her research, but also provides an excellent opportunity to look at practical and real world critical limitations in networks. The major activities conducted by the female PhD student so far include:
- Literature review to understand the field and find out the state-of-the-art
- Building theoretical framework for the QPON and remote powering scheme
- Perform numerical simulations and analysis of the theoretical framework
- Investigate the proposed remote power schemes, optimize the remote powering scheme on both power delivery and data communication aspects.
- Study the multiple optical pumps scheme to increase the amount of power that can be delivered to remote QPON without local power supply.
Due to the unavailability of suitable candidate, the undergraduate female student has not been involved in the stage 1 of the project. Fortunately, we managed to involve a female Master’s student in this project from the stage 2 of the project, so the student works on stage 2 and stage 3 of this project. The experience provided by the project to female students has enhanced the technical capability and showcased the concept of “women in engineering”, and we believe this can improve the gender equality in the engineering discipline in the future.
Project Communication Strategy
Since this project focuses on the initial study/verification of idea and the proof-of-concept demonstration, the targeted audiences are the related research communities in both academic and industry. Due to this nature, we have mainly communicated the key findings of this project via research seminars. Firstly, we have presented the key idea and preliminary findings of the project in our internal research seminar (School of Engineering, RMIT University). Initially we expected to attract our university industry partners to attend the seminar. However, due to the COVID-19, the research seminar has changed to the online virtual format, and only university students and faculty members can attend the seminar. The seminar has attracted over 20 attendance, and we have discussed the project idea and plan with interested students and academic staff. Secondly, we have also presented the findings of this project to other partners in other universities through invited research seminars. So far we have delivered two such research seminars, one at the University of Adelaide and the other at Nanjing University of Science and Technology, China. These research seminars have attracted over 80 attendance.
In addition, the key findings and outcomes of the project have been communicated via scientific publications. The paper, entitled “Remote Power of Rural Network Nodes for Future Optical Networks,” has been presented at the IEEE International Conference on Electronics and Communication Engineering (2021) [17], and has been published and accessible widely through IEEE. The presentation in the conference has attracted considerable attention, and has won the Best Paper/Presentation Award in the area of Mobile Communications Information Networks. Furthermore, to mitigate the miss of industry partner in the research seminar due to the impact of COVID-19, we also plan to present another research seminar in the face-to-face mode when allowed to attract industry partners.
For this industry-oriented seminar, we have planned to leverage with Wireless Innovation Laboratory (WiLAB), which is a multi-institutional collaboration involving the University of Melbourne and RMIT University, and it is new initiative and an innovation platform for next generation telecommunication technologies. WiLAB aims to enable frontier technologies to accelerate the update of next generation telecommunications in modern society, and it has already attracted a large number of industry partners. Therefore, through leveraging with WiLAB, the seminar will be made available to a much broader spectrum of audience, especially industry partners, to amplify the impact of this seminar and this project.
Recommendations and Use of Findings
The project has focused on the verification of the QPON and remote powering idea in networks and the comprehensive numerical simulation-based investigations. The outcomes of the project have given us strong confidence that the QPON and remote powering schemes are highly promising in solving the local power supply availability issue, which can better provide high-speed and reliable network access in remote communities in the future. Due to the elimination of the local power supply requirement, the findings of the project also have significant implication on the future network design, including the location of network nodes and the possible network topology to be used.
Based on the findings and outcomes of this project, we believe that QPON and remote powering have great potential. However, due to the idea verification nature of this project, we believe that there are still broad scopes/gaps to be filled before practical applications. Hence, we also summarize our recommendations for the next step as follows:
- In this project, the use of optical amplifiers is not considered, as the optical amplifiers require large power supply to realize the optical gain. However, in practical scenarios, particularly when the distance between network nodes becomes much longer, optical amplifiers may be needed and used. Hence, the impact of optical amplifiers on the remote power scheme needs to be further studied.
- In this project, we have focused on the impact of the strong optical pump on a single wavelength data channel. However, in current and future networks, the wavelength-division-multiplexing may be used to further boost the data rate. Therefore, the co-existence of multiple data signal channels with different wavelengths together with the strong optical pumps for remote powering needs to be further investigated.
- We have focused on the use of pulse amplitude modulation and the use of intensity modulation/direct detection in this project, which are widely used in optical access networks. However, when the distance becomes longer and with the development of technology, more complicated modulations involving phase modulation and the coherent detection may be used. Therefore, the use of QPON and remote powering technique is such networks needs to be further studied.
Bibliography
[1] Schrenk, B et al., “Passive optical switching engine for flexible metro-access,” Optical Communication (ECOC), 2014 European Conference on, 21-25 Sept. 2014.
[2] Supercapacitor. http://batteryuniversity.com/learn/article/whats_the_role_of_the_supercapacitor
[3] 2×2 MEMS OLS data sheet. http://www.sercalo.com/document/Datasheets/StandardSwitches/SX/SXLA_2x2.pdf
[4] A. Fallahpour et al., “Demonstration of Tunable and Reconfigurable Optical Nyquist Channel Aggregation of QPSK-to-16QAM and BPSK-to-4PAM using Nonlinear Wave Mixing and a Kerr Frequency Comb,” in Proc. Optical Fiber Communication Conference (OFC), 3-7 March 2019.
[5] D. Aguiar et al., “Automatic Tuning of Silicon Photonics Microring Filter Array for Hitless Reconfigurable Add–Drop,” Journal of Lightwave Technology, vol. 37, pp. 3939-3947, Aug. 2019.
[6] B. Zhang et al., “Optical filtering penalty estimation using artificial neural network in elastic optical networks with cascaded reconfigurable optical add–drop multiplexers,” Optical Engineering, vol. 58, pp. 076105, July 2019.
[7] Y. Wang et al., “Reconfigurable optical add-drop multiplexer at 1550 nm using magnetically-coupled switches based on a photonic crystal,” Optik, vol. 192, pp. 162878, Sep. 2019.
[8] J. Zhang et al., “Energy Efficient Baseband Unit Aggregation in Cloud Radio and Optical Access Networks,” Journal of Optical Communications and Networking, vol. 8, pp. 893-901, Nov. 2016.
[9] G. Talli et al., “Technologies and architectures to enable SDN in converged 5G/optical access networks,” in Proc. of International Conference on Optical Network Design and Modeling (ONDM), 15-18 May 2017.
[10] J. Wei et al., “Multi-band CAP for Next-Generation Optical Access Networks Using 10-G Optics,” Journal of Lightwave Technology, vol. 36, pp. 551-559, Jan. 2018.
[11] L. Neto et al., “Considerations on the Use of Digital Signal Processing in Future Optical Access Networks,” Journal of Lightwave Technology, vol. 38, pp. 598-607, March 2020.
[12] K. Inoue, “Four-wave mixing in an optical fiber in the zero-dispersion wavelength region,” Journal of Lightwave Technology, vol. 10, pp. 1553-1561, Nov. 1992.
[13] K. Markowski et al., “Influence of four-wave mixing in short- and medium-range 1310 nm dense wavelength division multiplexing systems,” Applied Optics, vol. 55, pp. 3051-3057, Apr. 2016.
[14] T. Salgals et al., “Investigation of 4-PAM modulation format for use in WDM-PON optical access systems,” in Proc. Progress in Electromagnetics Research Symposium (PIERS), 22-25 May 2017.
[15] J. L. Wei et al., “40 Gb/s Lane Rate NG-PON using Electrical/Optical Duobinary, PAM-4 and Low Complex Equalizations,” in Proc. Optical Fiber Communication Conference (OFC), 20-22 March 2016, pp. Tu3C.5.
[16] E. El-Fiky et al., “168-Gb/s Single Carrier PAM4 Transmission for Intra-Data Center Optical Interconnects,” IEEE Photonics Technology Letters, vol. 29, pp. 314-317, Feb. 2017.
[17] K. Wang et al., “Remote Power of Rural Networks Nodes for Future Optical Networks,” in Proc. IEEE International Conference on Electronics and Communication Engineering (ICECE), 17-19 Dec. 2021, pp. 261-265.
This work is licensed under a Creative Commons Attribution-ShareAlike 4.0 International License